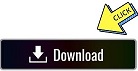

All images were processed with Adobe Photoshop 6.0 (Adobe Systems, San Jose, CA). Micrographs were obtained using a 63×/1.4 numerical aperture HCX PL Apo objective lens. Confocal images were taken using a Leica DM IRB microscope (Leica, Heidelberg, Germany) with a TCS SP3 AOBS scan head and equipped with argon and krypton laser beams.
Microvascular fragments meaning software#
Images were captured using Axiovision 4.3 software (Zeiss). Images of the cells were taken using an Axioplan 2 microscope equipped with an Axiocam MRm camera and a 40×/0.75 numerical aperture Plan Neofluar objective lens (Zeiss, Göttingen, Germany). Nonphagocytosed cells and fragments were then removed by intensive washing with ice-cold PBS and the cells were fixed in 4% PFA and embedded with Vectashield. When the cells reached confluence they were incubated with equal numbers of CMFDA-labeled apoptotic or necrotic HMEC-1 cells or with FITC-UEA-1–stained cell fragments for different time points. For some experiments HMEC-1 cells were labeled with the Chloromethylbenzamido Celltracker CM-Dil (Invitrogen) for 30 minutes. HMEC-1 cells were seeded on collagen-coated glass coverslips (12 mm in diameter) at a density of 4 × 10 5 cells. Sequence information of the oligonucleotides used for real-time qPCR is supplied in Table S1 (Table S1, available on the Blood website see the Supplemental Materials link at the top of the online article). 32 Primers were designed with Primer Express 2.0 software (Applied Biosystems). Data were analyzed using Q-gene software. qPCR was performed on a SDS 7700 system (Applied Biosystems, Darmstadt, Germany) with Rox dye as internal control (Invitrogen), FastStart Taq polymerase (Roche Diagnostics, Penzberg, Germany) and gene-specific primers in combination with SYBR-Green chemistry (Invitrogen) or with Fam-Tamra labeled TaqMan probes (BioTez, Berlin, Germany). For real-time qPCR, 2 μg total RNA was subjected to reverse transcription using a mix of random hexamers and oligo(dT) 12-15 oligonucleotides (Stratagene, Amsterdam, The Netherlands) and MMLV RNase H − point mutant reverse transcriptase (Promega). Quality of the RNA was determined using a Bioanalyzer and the RNA 6000 Nano LabChip (Agilent Technologies, Waldbronn, Germany). To obtain total RNA we used the RNeasy miniprep system in combination with an on-column DNase digest according to the manufacturer's protocol (Qiagen). These data indicate that CECs are not only markers of vascular damage but may induce proinflammatory signals in the endothelium. We therefore suggest that exposures to apoptotic and necrotic ECs induce different patterns of chemokine synthesis and leukocyte adhesion in healthy ECs. Both apoptotic and necrotic cells were bound and engulfed by HMEC-1 cells and primary human umbilical vein endothelial cells (HUVECs). Here we show that incubation of the human microvascular endothelial cell line (HMEC-1) with apoptotic ECs resulted in increased expression of chemokines and enhanced binding of leukocytes to HMEC-1 cells, whereas exposure of HMEC-1 cells to necrotic ECs caused no changes in leukocyte-binding affinity. The aim of this study was to evaluate the response of human endothelial cells (ECs) to apoptotic or necrotic ECs in an in vitro model and to delineate pathogenetic pathways.
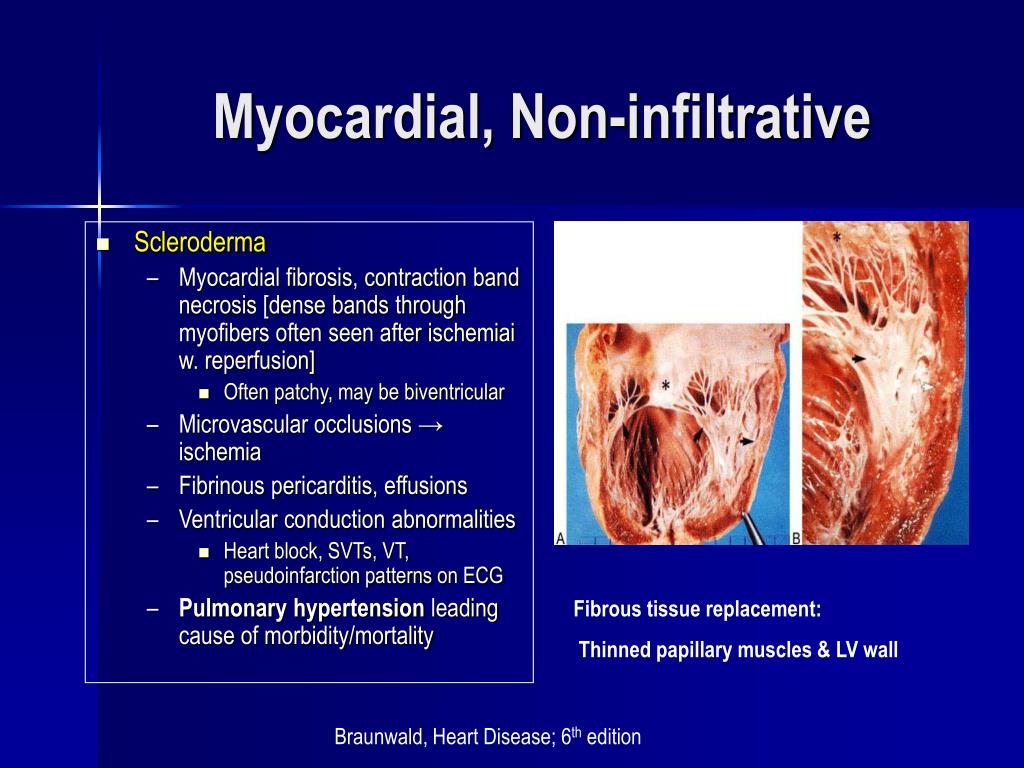
Circulating endothelial cells (CECs) have been detected in a variety of vascular disorders, but their interactions with healthy endothelium remain unknown.
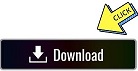